The Secret of Life
- Hermes Solenzol
- Jun 5, 2023
- 9 min read
How biochemistry unravels the mystery of life
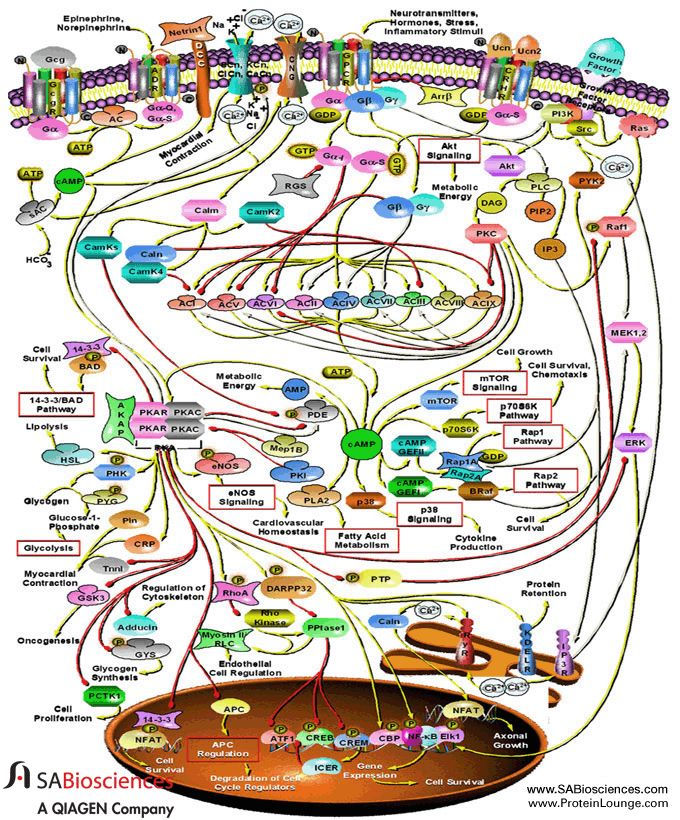
When I was in my last year of college, I had an epiphany. As I was listening to a lecture on thermodynamics of irreversible processes, my eyes were opened and I suddenly understood what is life. This was not some kind of mystical revelation but the culmination of two years of hard work studying biochemistry and molecular biology. But all of a sudden it all came together, and I understood what life is at its essence.
Vitalism
Since antiquity, people have wondered what makes living beings different from inanimate objects. The fact that an animal can be alive and then dead, and still look pretty much the same, gave rise to the idea of vitalism, which sustains that life is caused by a non-material essence, life force or Elan Vital, that infuses organisms with life and leaves them when they die. This idea persisted until the 20th century when it was debunked by the development of biochemistry, a science that explains life in terms of chemistry. But it is not as simple as that.
Schrödinger’s first hypothesis and the discovery of DNA
A good place to start is a book titled What is life? written by a famous quantum physicist, Erwin Schrödinger. The book came out of three public lectures that Schrödinger gave at the Trinity College, Dublin, in February 1943, while being exiled from Nazi Germany. In them, Schrödinger put forward two daring hypotheses.

The first, which he termed “order from order”, was a genial insight. It proposed that the genes used by living beings to pass information from one generation to the next were an “aperiodic crystal” that stored information as a code in its molecular structure.
He was right. The aperiodic crystal was found a decade later: it was DNA. An explosion of scientific discoveries followed. They established the genetic code and the mechanisms of transcription of DNA into messenger RNA (mRNA) and the translation of mRNA into proteins. These discoveries confirmed the theory of evolution by explaining:
the molecular identity of the genes,
the link between genes and sexual reproduction,
why genes last for many generations,
how changes in the DNA molecule are the mutations that drive evolution.
The gene-centric view of life
The problem was that many scientists fell so in love with genes and DNA that they thought that reproduction is the defining characteristic of life and the DNA-RNA-protein encoding system is its core mechanism. Everything else in a living organism is at the service of them. This view reflects the centralized and hierarchical way in which our society is organized. DNA is the king or the president. It sits in his throne in the nucleus at the center of the cell, from where it gives orders that are transmitted by its ministers, the messenger RNAs, and are faithfully executed by the workers, the proteins.
However, there are a few problems with the gene-centric view of life:
Reproduction is not the fundamental characteristics of life, because an organism can be alive even if it is unable to reproduce. Reproduction serves to perpetuate life in the long term and to drive evolution.
DNA is not essential to be alive. Red blood cells (erythrocytes) are alive even though they do not have a nucleus with DNA. In the lab, it is possible to keep alive cells without a nucleus or preparations like synaptosomes, presynaptic terminals pinched off neurons.
Viruses contain DNA or RNA, but they are not alive and able to reproduce until they infect a living cell.
How do we know that something is alive?
How do we know that red blood cells are alive and viruses are not? Because living cells breathe, consuming oxygen and producing CO2. They have an electric potential across their membrane. They can absorb substances from the outside and expel others. They consume substances to produce energy. All these properties mean that living cells have a metabolism.
Viruses do not breathe, have no membrane potential and do not move or consume substances. They have no metabolism.
To take a closer look, let’s consider what happens when a cell dies. To find out if a cell is alive, one of the things a scientist would do is to measure the amount of calcium ions (Ca2+) that it has inside. Living cells maintain a steep gradient of calcium concentration across their membrane, so that the amount of calcium inside is 10,000 times less than the amount of calcium outside.
Another way to know if a cell is alive is to look at the electrical potential across its membrane: it should be about -60 millivolts. This electrical potential is maintained by pumping sodium ions (Na+) to the outside and potassium ions (K+) to the inside, although their concentration gradient is not as dramatic.
A dead cell has a large amount of calcium inside and no membrane potential. All of its ion gradients have collapsed.
These are just two examples of things in a living cell that do not make sense from the chemical point of view. Things seem to be quite out of place. Ions should be equally distributed on both sides of the membrane. Unstable substances are present in large amounts. There is energy and chemical imbalance everywhere.
But what is even stranger is that, despite all that chemical imbalance, things stay the same. For example, the concentration of calcium inside a cell is always close to 100 nano-molar. Calcium concentrations may increase 10 times to convey a signal, but they immediately return to normal. Likewise, the concentration of any other substance within the cells is kept within a narrow range.
There is a word for this property of life: homeostasis. It means the ability of a living organism to maintain a stable internal environment.
Entropy and Schrödinger’s second hypothesis
Because chemical imbalances require energy to be maintained, to understand homeostasis we need to invoke some basics concepts of chemistry and thermodynamics. The second law of thermodynamics states that the amount of disorder in a closed system always increases over time. The precise term for disorder is ‘entropy’, which is a measure of all the possible configurations of a system. That means that a highly organized system, like a living cell, requires a lot of energy to stay that way.
Schrödinger also had an insight concerning the role of entropy in life. Unfortunately, it is not remembered as well as his prediction that genetic information would be stored in an aperiodic crystal. In his book What is Life? Schrödinger called his second hypothesis order from disorder. He said that for a living organism to keep its low entropy state it had to absorb energy and pump entropy to the outside. He called that property negentropy (negative entropy), but the term never caught on.
Homeostasis is a state far from chemical equilibrium
From the chemistry standpoint, increasing entropy means that a chemical reaction would proceed until the state of the lowest energy is reached. If compound A reacts with B to generate energy and compound C, the reaction will proceed until A and B are exhausted and all that remains is C. In other chemical reactions, compounds A and B react to produce compounds C and D, but the inverse reaction in which C and D produce A and B can also take place. In this case, an equilibrium is reached at some particular concentrations of A, B, C and D that minimize entropy. This is called chemical equilibrium.
A living cell is a vast network of interlinked chemical reactions. These reactions are constrained by barriers (membranes and the cytoskeleton) that create the external and internal structure of the cell. Remarkably, these chemical reactions never reach chemical equilibrium and, in fact, the concentrations of substances inside a living cell are far away from equilibrium. Still, their concentrations do not vary: this is what homeostasis means.
It is easy to keep concentrations of compounds the same at equilibrium, but to keep them stable far from equilibrium requires a system that carefully regulates the rate of those chemical reactions, speeding them up if the concentrations of products are too low and slowing them down if they are too high. This is achieved by having each reaction catalyzed by a protein called an enzyme, which is like a nanomachine with little control buttons that can speed it up or slow it down. So what presses those buttons? Well, other enzymes and signal molecules inside the cell.
DNA is not a king
The production of enzymes is regulated by the DNA-mRNA-protein genetic mechanism that I described above. But the DNA is not the king but just another piece of the machinery. DNA does not give orders, it follows them. And who orders DNA around? Proteins and small signaling molecules that tell the DNA which genes get to be expressed and how fast. So proteins are not the proletariat of the cell, but they are not kings, either. They follow orders from other proteins.
Living cells are not organized like our societies or even our computers, because there is no command center. The cell just keeps going by having its parts interact which each other and with the environment.
The best way to understand this is to view the cell as an information processing system, computing endlessly the best way to keep its homeostasis going. If I were to use a computer metaphor, DNA would not be the central processing unit but the hard drive where information is stored long term and extracted when needed. And it is a read-only hard drive, because permanent changes in the DNA genetic information are not allowed. Only the twin processes of mutation and natural selection get to make permanent changes in the DNA.
Prigogine, dissipative structures and intracellular signals
The idea that a living system is a system far from equilibrium or, as he called it, a ‘dissipative structure’, was envisioned by Ilya Prigogine, who wrote mathematical equations explaining this behavior. Recent discoveries on cell biochemistry supported his ideas. They unraveled the complex networks of intracellular signals that maintain homeostasis. Not only that, they also explained how a cell reacts to its environment or signals from the rest of the body, if the cell is part of a multicellular organism.
Systems that are far from equilibrium and yet keep their shape are all around us. For example, a river: the water that flows in it is never the same and yet the river keeps its shape. The river is part of a non-equilibrium system: energy from the sun evaporates water from the sea that rains in the mountains and flows back to the sea, forming the river. But while the river may be turbulent and uneven below its surface, in a living cell every molecule is precisely placed and controlled. A more apt metaphor for a living cell will be ten thousand people juggling balls and passing them to each other in a precisely coordinated way.
How did life get started?
The homeostatic view of life has profound implications in many fields of biology. Take the problem of the origin of life, for example. The most accepted view these days is that of the RNA-world, in which molecules of RNA interacted with proteins to catalyze the first reactions of life. However, before that, there had to be a long process in which chemical reactions became progressively interlinked to create the first self-sustained entities. Then a primitive form of natural selection favored the most stable of those entities, slowly encouraging internal control to finally giving raise to homeostasis. The tightly regulated homeostasis of modern organisms probably took a lot of trial and error to evolve. With it came the formation of proteins, catalysis of reactions by proteins or RNA and, finally, DNA and the genetic code.
Life as information processing
That day in my last year of college, while the professor explained Prigogine’s ideas, it all came together. I understood that life is really homeostasis.
Over the years, as I studied cell signaling systems with my own experiments, I realized that there is something beyond homeostasis. What keeps the system together in all its delicate balance is the flow of information through its signaling systems. Although there is no central processing unit, there is a certain hierarchy of control. “Intracellular signaling systems”, as they are called, form pathways for the transmission, amplification and processing of information. For example, one of them is formed by the enzyme adenylyl cyclase, the small molecule cyclic-AMP and enzyme protein kinase A, which changes the functioning of many proteins by attaching phosphoric acid to them in a process called phosphorylation. Other enzymes, the phosphatases, remove the phosphate. But there are many other signaling pathways, forming all kinds of complicated branches and loops. We are still trying to classify them and understand them.
Its essence, then, life is the processing of information with the goal of keeping itself going. Reproduction is just a way to prolong the process in the long term, thanks to the effectiveness of DNA in preserving information.
A living organism, therefore, is something essentially different from a material object. An object keeps its shape because its atoms and molecules stay in the same place. Inside a living organism everything moves; matter and energy come in and out. Like the water that flows in the river, atoms are not there to stay, they are just passing by. What always remains, in the end, is the information.
Comments